In terms of muscle mass and enhanced sports performance, and following on from Part I, Robuvit intake has also been linked to improved protein production because of increased ribosome biogenesis.1
Ribosomal biogenesis is vital for protein synthesis and cellular functionality. These findings suggest that Robuvit has the potential to strengthen the body’s adaptive response to challenging situations by providing an appropriate amount of protein and to enhance physical endurance and fitness.
In subsequent clinical studies, improved performance during sportive activities, such as triathlon or trekking, was observed after Robuvit supplementation.22,25,26
Fifty four recreational athletes in their thirties trained together for a triathlon for 2 weeks.25 Half of them took Robuvit every day; the other half was assigned to a control group.
After the 2-week training and supplementation phase, a performance increase was seen in all subjects in the triathlon.
The time needed for the respective disciplines was significantly reduced in the Robuvit group: 12.35 minutes for swimming (–15% versus baseline), compared with 13.18 minutes in the control group (–7%), 34.04 minutes for cycling (–9%), compared with 35.73 minutes in the control group (–1%) and 23.47 minutes for running (–12%) compared with 24.63 minutes in the control group (–4%).
In addition, both muscle pain and post-performance cramping were significantly lower in the Robuvit group compared with the control group. Likewise, the time to fully recover was reduced by 10.4% with Robuvit.
Interestingly, lactate dehydrogenase and unconjugated bilirubin were significantly increased in the control group after the event, but not in the Robuvit group. These markers of haemolysis suggest a state of exercise-intensified oxidative stress, which was kept at bay with Robuvit supplementation.25,27
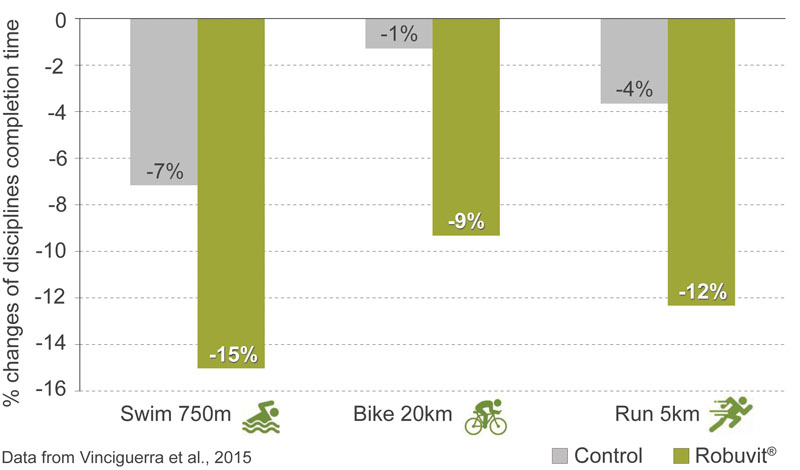
Figure 2: Robuvit improves triathlon times after 2 weeks
Oxidative stress can also influence ribosome biogenesis, potentially leading to cardiovascular, neurodegenerative and skeletal disorders.1,28
Given that Robuvit not only stimulates ribosome biogenesis but also exhibits antioxidative effects, it helps the body to adapt to challenging situations more effectively and quickly.1,18,23,29
Clinical studies have shown that Robuvit lowers plasma oxidative stress and increases the levels of antioxidant enzymes.1,18,23,29
A 1-month clinical study involving 20 healthy volunteers investigated the antioxidant potential of Robuvit.29 The results showed a significant decrease in advanced protein oxidation products in the blood by more than 40% and lipid peroxides by more than 20%.
This shows that Robuvit protects proteins and lipids from oxidation. Moreover, the study revealed that Robuvit supplementation significantly stimulated the presence of enzymes that protect the cells from oxidative attack, such as superoxide dismutase and catalase.29
Finally, Robuvit significantly increased the plasma antioxidant capacity assessed by the FRAP (Ferric Reducing Ability of Plasma) assay. These findings suggest that Robuvit can reduce oxidative stress and enhance the body's antioxidant defences.
Interestingly, the study also found that the effects of Robuvit persisted even after suspending its intake. Two weeks after supplementation, the levels of oxidised proteins and lipid peroxides remained significantly lowered.29 This indicates a lasting impact of Robuvit on oxidative stress markers.
Another double-blind, placebo-controlled study in patients recovering from surgery confirmed the antioxidant effects of Robuvit.18
After 4 and 8 weeks, the levels of oxidised proteins and lipid peroxides were significantly lower in the Robuvit group compared with the placebo group. This supports the previous findings and further validates the antioxidant properties of Robuvit.
Supported liver function and detoxification
The third gut metabolite found in blood following Robuvit intake, urolithin C, has been the subject of research regarding its potential in terms of liver health.
Urolithin C was investigated as an inhibitor of liver pyruvate kinase, the inhibition of which could be beneficial when it comes to halting or reversing non-alcoholic fatty liver disease.1,30,31
In an experimental study, the oral administration of urolithin C showed protective effects against liver damage and improved various markers of non-alcoholic fatty liver disease.32
The study suggests that the activation of the hepatic AMPK (AMP-activated protein kinase) signalling pathway plays a role in the beneficial effects of urolithin C on non-alcoholic fatty liver disease.32
Liver cells possess the highest concentration of mitochondria in the human body and mitochondrial dysfunction has been involved in liver injury, including alcoholic and non-alcoholic fatty liver disease and liver inflammation.33
Thus, Robuvit’s enhancing effects on mitochondrial function and its ability to inhibit liver pyruvate kinase contribute to overall liver health.10,11,17
In a 3-month clinical study, Robuvit was found to improve liver function and help prevent liver fibrosis progression in people with early symptomless hepatic damage from non-alcoholic fatty liver disease.17
The increased serum levels of two markers for non-alcoholic fatty liver disease decreased significantly with Robuvit compared with controls. Likewise, platelet count and albumin levels improved significantly with Robuvit.
The risk for liver fibrosis decreased significantly with Robuvit supplementation, compared with controls, according to the decreased APRI (Aspartate Transaminase to Platelet Ratio) Index.
After Robuvit supplementation, a liver ultrasound showed a significant decrease in the size of the liver and a lower echogenicity, representing fewer fibrotic changes.
Another “detox effect” was observed after supplementation with Robuvit in several studies: an amelioration of lymph drainage.34–37
The mechanism of action of this effect is not yet fully elucidated but the antioxidative properties of Robuvit have beneficial impacts in this context; high oxidative stress, the enhanced formation of reactive oxygen species and accelerated lipid peroxidation processes are present in chronic lymphoedematous tissue.38
The effects of Robuvit on early lymphatic disease were investigated in a study with 32 women.36 Robuvit intake led to decreased leg volume by 16.4±1.1% compared with a decrease by 9.3±1% in controls after 4 weeks.
In addition, the oedema score, the interstitial fluid/plasma protein concentration ratio, limb size and ankle circumference decreased significantly with Robuvit. These effects show Robuvit’s potent efficacy for improved detox activities in the body.
Summary
Robuvit French oak wood extract helps to improve energy and performance, enhance detoxification and reduce fatigue. These effects were shown in clinical studies and are based on well-investigated mechanisms of action.
Robuvit has been shown to have strong antioxidant activities, improve mitochondrial rejuvenation and the mitochondrial respiratory chain, thereby optimising ATP generation, and has beneficial effects on muscle tissue synthesis and liver health that help to explain its observed benefits.
References
25. M.G. Vinciguerra, et al., Minerva Cardioangiol. 63(5), 403–409 (2015).
26. G. Belcaro, et al. Minerva Med. 109(6), 451–456 (2018).
27. G. Lippi, et al., Annals of Translational Medicine 7(12), 270 (2019).
28. A. Pelava, et al., Biochem. Soc. Trans. 44(4), 1086–1090 (2016).
29. M. Horvathova, et al., Oxid. Med. Cell. Longev. 2014, 639868 (2014).
30. U.M. Battisti, et al., Pharmaceuticals 16(5), 668 (2023).
31. U.M. Battisti, et al., Nutrients 15(3), 577 (2023).
32. J. Xu, et al., Naunyn Schmiedebergs Arch. Pharmacol. 396(10), 2687–2699 (2023).
33. P. Middleton, et al., Therap. Adv. Gastroenterol. 14, 17562848211031394 (2021).
34. G. Belcaro, et al., Int. J. Angiol. 24(1), 47–54 (2015).
35. G. Belcaro, et al., Minerva Chir. 73(3), 288–294 (2018).
36. S. Hu, et al., Panminerva Med. 65(1), 91–92 (2023).
37. S. Hu, et al., Minerva Cardioangiol. 68(3), 197–202 (2020).
38. W.G. Siems, et al., QJM 95(12), 803–809 (2002).